- The Daily Meathead
- Posts
- Biceps eBook Free Sample
Biceps eBook Free Sample
Below is a free sample of the first 3 sections of my biceps web eBook, which contains over 15,000 words, 60+ diagrams, exercise tutorials & more.
Introduction
The goals of this ebook are very simple:
Learn everything about the biceps & other elbow flexors, their anatomy, and the joints they act on.
Learn how to train them.
That’s it.
For every fancy-shmancy anatomy term, phrase, or complete thought you read, I will provide a layman’s English translation. This is because many of you are likely in very different stages of learning. Many of you also may not have an interest in understanding jargon at all. To accommodate all kinds of people and the associated learning phases, I will try to keep both the nerdy and simplified explanations concise and coherent without missing key details.
Our journey in understanding the elbow flexors begins with a discussion of muscle architecture. In this section, we’ll discuss everything you need to know about where the elbow flexors attach, what their unique properties as a muscle group are, and what their function is as a consequence.
Muscle Architecture & Basic Function
You probably purchased this eBook because you read “biceps”, and not because you thought about the “other" elbow flexors in the description (the other muscles that bend the elbow along with the biceps).
In any case, it’s important to discuss the other elbow flexors in addition to the biceps because we cannot separate their functions when it comes to resistance training.
If you bend the elbow against resistance, all of the tissues that bend the elbow will respond, including the ones that people rarely talk about.
As we’ll learn in the following sections, these “other” flexors are just as important to the function of the biceps as the biceps themselves (yeah, it sounds crazy, but just trust me on this one).
The Biceps
The biceps brachii are a group of two distinct muscles (referred to as “heads”) which attach on the front side of the shoulder and forearm.
Long head of the biceps - attaches from the supraglenoid tubercle and shoulder labrum down to the radial tuberosity and bicipital aponeurosis.
Translation: attaches from the shoulder blade down to the front of the forearm bone and a flat tendon that sits on top of forearm tissues.

Credit: Complete Anatomy
Short head of biceps - attaches from the tip of the corocoid process down to a similar location as the long head.
Translation: attaches from the shoulder blade down to (roughly) the same location as the long head of biceps.

Credit: Complete Anatomy
Both of the biceps are fusiform muscles, which mean that their fibers are aligned parallel to one another inside of each muscle belly (see above).
Both of the biceps attach to the scapula directly and therefore play an important role in stabilization of the shoulder joint. Because the biceps do not share attachment sites at the shoulder, they have the potential to play different roles depending on joint position.
Both of the biceps attach to the radius (one of the two bones in the forearm complex), which gives both of them the ability to flex (bend) the elbow and supinate the forearm.
This technically makes each of the biceps a three-joint muscle, meaning that each of them have the potential to influence motion at three separate joints (shoulder, elbow and forearm).
If you're not familiar with terms like elbow "flexion" and forearm "supination", here's a visual reference for what both look like:
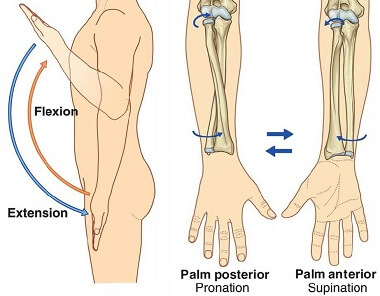
Image credit: https://www.shoulder-pain-explained.com/
For a long time it was believed that both heads of the biceps shared a common insertion on the forearm, but recent studies have challenged this belief.
The short head of the biceps appears to attach at a slightly more distal portion of the radius as compared to the long head. Both heads still consistently appear to converge into the bicipital aponeurosis (the flat tendon in the photos above).
Translation: the short head of the biceps attaches farther away from the elbow than the long head in some individuals. Whether or not this has a high degree of implication in a resistance training setting is unclear. Below is a photo of a case wherein both biceps do not share a common insertion:

Credit: van den Bekerom MP, PMID: 25231429.
A number of different studies have also shown that, in a minority (2-3%) of the population, the biceps can have anywhere from 3-7 heads as opposed to the typical 2.
The roles that the short and long biceps play at the shoulder are substantial in their influence but minimal in motion. What does that mean?
Both of the biceps “cross” the shoulder, but neither are capable of creating rotation of the upper arm to the extent that our other shoulder muscles are.
This is specifically because of where the biceps attach and how their attachment sites dictate the lines of tension (known as action lines) they create.
The long head of the biceps attaches directly above the head of the humerus on the scapula and is continuous with the glenoid ligament in many people. It wraps around the bone like a cable wraps around a pulley.
Because of this, its function at the shoulder is similar to the “rotator cuff” muscles of the shoulder insofar as they compress (“smush”) the joint space together.
Note how, in the diagram below, the net action line of the long head - in this position - is directed as a straight line into the socket of the scapula.

Credit: Complete Anatomy
This function of the long head of biceps is well-documented in research. Because of how close to the center of the shoulder joint it sits, it does not function as a “flexor” of the shoulder as many textbooks commonly cite.
Broad descriptions of motion are helpful for understanding anatomy generally but substantially limit our ability to be precise about which muscles perform which actions in different positions. This kind of "textbook confusion" plays a disproportionate role in poor exercise selection and execution.
The short head of the biceps, also having an attachment on the scapula, creates force across the shoulder joint.
The nature of its torque on the shoulder is distinct from the long head of biceps because it’s attachment sits more medially and does not wrap around the head of the humerus.
Translation: the short head acts very differently at the shoulder because it attaches farther in on the scapula and does not wrap around the head of the upper arm like the long head does (refer to the photo above).
As a consequence of this distinction, the short head of biceps has a greater capacity to produce rotation at the shoulder than the long head. These differences will have implication when it comes to applying anatomy to lifting, which will be discussed in the resistance training section.
The “Other” Elbow Flexors - Brachialis & Brachioradialis
The brachialis and brachioradialis are single and two-joint muscles, respectively.
The brachialis attaches from the anterior aspect of the middle of the humerus and attaches to the ulna.
Translation: the brachialis attaches from the upper arm down to the forearm bone that doesn’t spin.

Credit: Complete Anatomy
The brachioradialis attaches from the lateral distal humerus down to the styloid process of the distal radius.
Translation: the brachioradialis attaches from the bottom of the upper arm down to the bottom of the forearm near the wrist.

Credit: Complete Anatomy
The brachialis, being a single joint elbow flexor, is incapable of any other function. This means that, whenever we bend the elbow against resistance, the brachialis must contract. There is no way around this.
The brachioradialis, on the other hand, traverses across the forearm and can rotate the forearm complex.
The brachioradialis is unique because, in addition to being able to bend the elbow, it can supinate (like the biceps) from a pronated position and pronate from a supinated position. Both of these occur in tandem with the elbow flexion that it creates.
This is defined as a "reverse action" property of a muscle. Refer to the image above on pronation/supination if those joint actions are visually unclear.
So while both the brachialis and brachioradialis play a significant role in bending the elbow, the brachioradialis is affected directly by position of the hand and how we relate to the direction of external resistance. This will have implications insofar as resistance training is concerned, which we will cover in a later section.
Joint Structure & Mechanics
In order to understand function and leverages of the different elbow flexors and how they may differ, a deeper understanding of the elbow and forearm complex is necessary.
The elbow (humeral-ulnar) joint is a hinge joint, which means that it only moves in one cardinal plane of motion - the sagittal plane.
Translation: the elbow joint just bends and straightens around a single pivot point, much like a hinge on a door.
The elbow joint itself is separate from what most perceive to be elbow-related motion - the elbow itself describes the contact relationship between the proximal portion of the ulna and radius and the distal portion of the humerus. Many have described the elbow as the connection between the proximal radius and ulna, but this is a separate joint known as the proximal radio-ulnar joint (which allows for forearm rotation). There are a number of sources that may disagree with this, but in my estimation, it is best to separate the discussion of the elbow from the forearm in this way for ease of understanding.
Translation: the elbow is the connection between the forearm bones and the upper arm. The elbow does not generate the twisting motion that one can create within the forearm, only the bending motion that occurs between upper arm and forearm.
In our discussion of elbow mechanics from here forward, whenever the term “elbow” is used, it is used to describe this bending motion only. The forearm and its motion are of significance but will be referred to separately and specifically moving forward.
The image below - which you've already seen - displays elbow motion (left) versus forearm motion (right). These are not mutually exclusive motions.
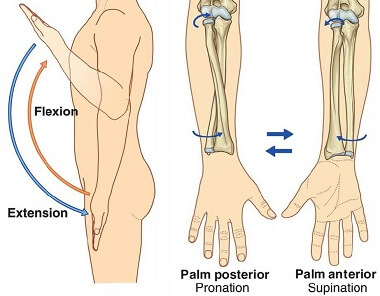
Image credit: https://www.shoulder-pain-explained.com/
Motion of the elbow is akin to motion of a wrench around a bolt - the wrench can only effectively turn in a single direction, and thus muscles that act around this “wrench” can only be substantially loaded when the forearm is loaded in accordance with this.
Imagine attempting to turn a wrench in a direction that was 90° from the direction that a bolt would spin - the wrench would either detach from the bolt, or the bolt itself would be pried out of whatever socket it was being screwed into.

Image credit: Darling Kindersley (above), https://musculoskeletalkey.com/ (below)
When the elbow is loaded with an external force that directs the forearm or upper arm in a side-to-side manner, the passive structural elements of the elbow - such as the surrounding ligaments and bony contacts between the upper arm and forearm - play a relatively larger role in stabilization.
Translation: when the elbow is loaded sideways in the direction it does not move, non-muscle (connective) tissues primarily stabilize the joint.
Supination and pronation are motions that occur specifically in the forearm around an axis that runs through the radius bone - not the elbow - which occur as a consequence of the relationships between:
The proximal radius and the distal humerus.
The proximal radius and ulna.
The distal radius and proximal carpal bones.
The distal radius and ulna.
Translation: supination and pronation are the “twisting” motions that occur when the radius folds around the ulna. This is the motion that occurs when your hand looks like it's twisting. Imagine you’re standing upright with your elbow bent to 90º - supination would be turning your palm to the ceiling, and pronation would be turning your palm toward the floor. Some folks remember this well when they visualize standing upright with a bowl of soup (like “soup”ination - hah!) and pronation as spilling the soup on the floor.
As a reminder (nail this into your head please):
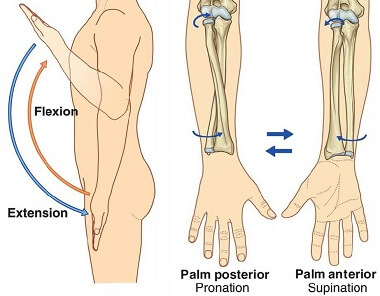
Image credit: https://www.shoulder-pain-explained.com/
Under “normal” circumstances, the elbow bends and straightens a total of ~150º of motion, with exceptions on either end of the spectrum into hyper-extension of ~0-10º (straightening beyond “straight”) and passive bending beyond ~150º of an additional ~10-20º (wherein non-contractile tissues control motion and where external forces provide the motion).
In addition, greater amounts of elbow flexion (bending) are observed in a supinated position, whereas greater amounts of elbow extension (straightening) are observed in a neutral or pronated position. This is due to the length change and contractile ability of the biceps as well as the relationship between the bones of the forearm and the upper arm.
The elbow presents with a “normal” valgus of between ~10-15º in men and an additional ~5º in women. This appearance and the average range of motion has the capacity to alter substantially based on multiple factors:
Muscle bulk in the forearm, upper arm and trunk.
Available range of motion and joint position of the forearm (which, under “normal” circumstances, falls anywhere between ~85º of supination and ~75º of pronation).
Whether the motion observed is passive or active - i.e., whether one is actively contracting against resistance or whether one is being moved passively into a position.
These ranges and positions - like in many other joints - have the capacity to alter dramatically based on the above variables. It is therefore unhelpful to generalize joint motion beyond this as motion is highly context-dependent upon imposed internal and external forces.
Translation: the elbow can typically bend anywhere from -10º of straightening to 170º of bending depending on the context-specific forces and forearm position. The forearm appears at a greater angle outward when in anatomical position as compared to when the elbow is bent. This is called a carrying angle, and is normal between 5-15º. A visual reference:

Image Credit: Iyer, K.M. (2020). https://doi.org/10.1007/978-3-030-43286-7_4
The elbow is considered a stable joint relative to other kinds, such as ball-and-socket joints like the shoulder and hip. This is because the elbow joint can only bend and straighten in one direction and as a consequence is highly supported by structure.
A good rule of thumb - in general - is that joints that possess more movement options - like the shoulder complex - are less structurally stable but can move more as a consequence. Joints that have fewer movement options, like the elbow, are more structurally supported because they cannot move as much.
You might at this point be asking - Ben, why the hell do I need to know about all this bone stuff? I thought we were going to talk about how to train my biceps! And you’d be justified in that thought. But let me provide you with a different perspective that may blow your socks off…
People often look at muscle first when it comes to understanding anatomy. And I get that. Muscle is flashy, and it's the stuff that everyone wants more of.
But to understand the elbow flexors, their anatomy and how to train them, we need to understand the structure that creates the foundation for their function to begin with.
Think about it this way: if you were going to try to build a house, you’d first need to design a blueprint for its foundation. You might imagine all of the rooms you’d want to have, what function each of them would serve, and what you’d do in each room, but none of that could ultimately manifest if you didn’t understand how to build the foundation of the house and how to connect each floor.
The relationship between bones, ligaments, and muscles ultimately works the same way. Understanding muscles is nice - but you can’t understand muscles without understanding what they’re built to serve in the first place - our joints, and the bones that create them.
Hopefully I’m making sense. Let’s move on.
Elbow Flexor Mechanics
The mechanical ability of each of the elbow flexors in bending the elbow is quite different. Analyzing which flexors perform best in what positions requires an understanding of action line and it’s relationship to different joint positions.
An action line of a muscle represents which direction a muscle pulls a bone in a given joint position.
Action lines ultimately represent the direction of tension in a muscle and tells us what that muscle is “trying” to do to a set of bones.
To draw an action line, we must look at a joint 90° from the potential direction of motion. Reasons for this will become more clear as you read.
When analyzing elbow motion, this means looking at an angle from the perspective of the photo below (technically known as the sagittal plane of the joint):

Credit: Complete Anatomy
Pictured above are all of the elbow flexors, with the biceps shaded in gold.
To draw an action line, identify the tendon of the muscle in question and its location and direction of pull on the relevant bone(s). Because we are only looking at elbow motion and forces in this context, we can draw the action line of both of the biceps from the forearm upward:

Credit: Complete Anatomy
Note how the action lines - represented by the green arrows - extend in a straight line upward from the tendon of the distal biceps.
Drawing action lines is distinct from depicting what direction that individual fibers of a muscle are doing.
For example, many of you may have seen a depiction of a similar scenario which looks like this:

Credit: Complete Anatomy
This wouldn't necessarily be "wrong" in an absolute sense, but would indicate the individual lines of net tension that the muscle (in and of itself) are creating. This is an accurate depiction of what the biceps are "trying" to do when it contracts, but these are not representations of how the biceps act on the bones they attach to.
When our goal is to analyze function of a muscle and the "leverage" that it has to perform specific joint actions, we cannot utilize the strategy above. This is because these are not action lines but are more broad, non-technical ways to look at how a muscle creates tension in and of itself.
So, when analyzing joint forces and motion specifically, we must draw action lines from a muscle's last point of contact on a bone.
This means means that we draw an arrow in a straight line from the muscle's tendon, in-line with how the tendon pulls on the bone directly.
Let's now look at the action lines of the brachioradialis in the two joint positions above:

Credit: Complete Anatomy
Notice how distinct these action lines can be from one another in terms of the joint forces and motion that they have the potential to create.
In the bottom (more elbow-straight) position, the brachioradialis creates a force that can bend the elbow and that can "smush" the joint space together (technically known as joint compression).
In the top (more elbow-bent) position, the brachioradialis creates a force that mostly just "smushes" the joint spaces together.
In other words, the brachioradialis transitions from being able to create substantial amounts of elbow bending to mostly just acting as a "joint stabilizer". This does not mean that its role is any less or more important, but rather that its function at the elbow joint across a variety of positions changes substantially.
Let's now look at the action lines of the brachialis in the same positions:

Credit: Complete Anatomy
In either position, the function of the brachialis remains an "elbow bender". In terms of the relationship between joint positions, though, it is fair to say that the brachialis has a stronger ability to bend the elbow in a more elbow-bent position because of how it changes relationship to the forearm and upper arm.
If these depictions are at all confusing, here's a more principle-based approach to identifying what role each elbow flexor plays in either of the joint positions above (as well as any other position in between these two):
First, draw the action line as previously instructed. Make sure that it is an arrow that extends in a straight line from the tendon of the muscle where it attaches on the forearm. Because this line indicates a direction of influence, you can extend it to "infinity".
Next, look at the relationship between the action line and the bones. If the action line has a perfectly parallel relationship to the forearm complex, then you know that it doesn't contribute much to rotation of the elbow, but rather compression (or distraction, which is the opposite) of the joint surfaces. If the action line has a perfectly 90º relationship to the forearm complex, then you know that it doesn't contribute much to compression (or distraction, the opposite) of the joint surfaces, and rather contributes far more to rotation of the elbow.
In my experience teaching, many folks will get caught up in attempting to draw moment arms first (which we will do next), but this more often muddies the water. If you understand the relationship between the relevant bones and action lines, moment arms are icing on the cake.
Let's now look at each of the elbow flexors at three separate points of elbow rotation individually, so that we can really hone in on what each one has leverage to do throughout the range of elbow bending. In the following images, I'll indicate the action line and moment arm of the specific muscle in question.
A moment arm is simply a representation of a muscle's rotation-producing capability in any position. It is an imaginary line we draw to gain an understanding of where a muscle will have more or less of an ability to rotate a bone. It is technically defined as the horizontal distance between a force and a pivot point. If that's confusing to read, it'll be much easier to understand with the visuals below.
Starting with the biceps...
Position #1 - minimal elbow bending:

Credit: Complete Anatomy
Note how the moment arm (blue) is drawn exactly 90º from the action line and how it intersects with the pivot point (elbow). Recall that this is not a tangible distance but rather an imaginary line that we use to represent how much leverage a muscle has in a given position.
Recall the principles that we just went over. Look at the relationship now between the green action line and the forearm bones. Is it parallel in this case? No, but it's not far off.
What this means is that the biceps create both rotation and joint compression in this position (picture how that action line would "smush" the forearm into the upper arm, like you're grabbing both bones and squeezing them together).
An important aside on moment arms: the absolute length of each moment arm we depict in and of itself is not relevant. Moment arm length is only relevant in how it relates to other moment arm lengths. In other words, what is important in understanding resistance training is not the precise length, but how moment arms of other muscles relate to the one in question in any given joint position.
Position #2 - mid-range elbow bending:

Credit: Complete Anatomy
Note how much longer the moment arm is in this position as compared to the first. What does this imply?
The biceps now have a much more leverage to produce rotation at the elbow as compared to the first position. Notice that I did not say that they would necessarily be stronger in this position, which is something we'll cover in the research section (as a teaser: how "strong" a muscle is is a combination of the leverage we're talking about now as well as the length of a muscle).
Also note the action line's relationship to the forearm complex. What do you see?
In my estimation, the action line is almost perfectly 90º from the forearm complex here, which means that almost all of the tension in the biceps at this point are contributing to rotation of the elbow as opposed to compression or distraction (recall that distraction is technically the opposite of compression).
Position #3 - end-range elbow bending:

Credit: Complete Anatomy
Recall earlier how I mentioned that an action line indicates a direction of pull? This means that we're able to extend it infinitely in both directions. In this case, extending the action line downward is necessary so that we can intersect the action line with moment arm to the elbow.
The moment arm in the end-range elbow-bent position is more similar to the mid-range position than the initial (elbow-straighter) position we looked at. We can identify this through observation of moment arm (blue) as well as the relationship between action line and forearm complex.
Something interesting happens when the elbow is bent more than 90º.
In the initial (non-bent) elbow position, the biceps create a small amount of rotation and a substantial amount of joint compression (think: bones being "smushed" together).
In the final (elbow-bent) position above, the biceps create a substantial amount of rotation, but now create a joint distraction force.
Why?
Because the biceps have an action line that interacts with the forearm at a non-90º angle, but now the action line pulls the forearm "away" from the elbow instead of "smushing" it together.
But there's a catch....
A VERY important note on distraction: earlier, we defined distraction as the opposite of compression. This may lead you to believe that distraction is bad and compression is good. You might think that compression holds your joints together and that distraction pulls them apart.
From a non-contextualized perspective, you'd be correct. Distraction is pulling surfaces apart and compression is the opposite. BUT distraction and compression are always joint and position-specific terms that need to be looked at in individual scenarios.
In the image above, many textbooks will describe the biceps as creating a distraction force at the elbow, and they leave it at that. Again, this leads people to think "bad" because of "joint separation".
In reality, if you look at the structure of the elbow - recall the earlier section on its structure and how stable it is - a "joint distraction" force in the position above would simply "smush" the back side of the elbow together.
So, in reality, distraction in this specific force scenario is just code for compression on the other side of the joint. This is why all joint force descriptions need to be specific to a given context and not used in generalization.
To summarize the above three phases of elbow flexion:
Position #1 - biceps have the least amount of relative leverage to rotate the elbow (although they still have some) and mostly "smush" the elbow together (compression of the bottom side of the elbow).
Position #2 - biceps have the highest degree of relative leverage to rotate the elbow and very little (if any) to compress or distract the joint surfaces.
Position #3 - biceps have a combined ability to rotate the elbow (more than position #1, less than #2) and an ability to distract the joint surfaces (read: compress the back side surfaces of the elbow together)
Moment arm for the biceps is longest somewhere in the mid range and drops off substantially toward the more elbow-extended position
Let's look into the brachialis next.
Position #1...

Credit: Complete Anatomy
Similar to the biceps, the brachialis has a relatively smaller moment arm in the more elbow-straightened position above. The brachialis contributes substantially to joint compression here, given that its action line is closely aligned parallel with the bones of the forearm.
Position #2...

Credit: Complete Anatomy
The brachialis gains leverage via a substantially increased moment arm in the mid-range position. Note how the action line of the brachialis is close to 90º from the forearm, much like the biceps in this joint position (the biceps are faded here, but you can still see their tendon and its theoretical action line). This means that the brachialis mostly contributes to rotation in this position rather than compression or distraction.
Position #3...

Credit: Complete Anatomy
Much like we had to do with the biceps, the action line extends downward so that moment arm to the elbow has a capacity to intersect action line.
The brachialis creates similar forces to the biceps in this position, meaning that it continues to create rotation but transitions from compressive to distractive forces above the mid-range of elbow bending. Again, recall that this is not actually a true distraction force, but rather a compression force applied to the opposing side of the elbow.
The summary of positions, force types and their relative moment arms are the same as the biceps above. Interestingly, the brachialis is kind of like a mini-biceps in this regard. More will be discussed in this resistance training section on how you might go about targeting these muscles differently.
Lastly, let's look at the brachioradialis.
Position #1...

Credit: Complete Anatomy
The brachioradialis has a moment arm for rotation that is substantially longer than both the biceps and brachialis from this elbow-straightened position. Although these depictions are crude and not perfectly to size, this is a quite obvious distinction that can be made across any anatomical model.
The brachioradialis also serves to compress the joint space at this point, given that its action line is only 10º or so from being parallel to the forearm complex. Despite this, the brachioradialis has a substantial ability to produce rotation at the elbow in this position, given how long its moment arm is in comparison to the biceps and brachialis.
As an aside: because this force analysis is only done from a single perspective, there may be complicating factors in terms of what ends up "recruiting" the brachioradialis more so than the other elbow flexors - such as forearm position and rotation - but this will be discussed in the resistance training section.
Position #2...

Credit: Complete Anatomy
Although its moment arm still allows for some degree of rotation, much of the tension in the brachioradialis in this position is dedicated to smushing the joint surfaces together.
This is apparent due to the fact that its action line is nearly parallel to the bones of the forearm and that the moment arms of both biceps and brachialis are substantially larger than they were compared to the elbow-straightened position.
We will return to the discussion of this position in more depth in a little bit.
Position #3...

Credit: Complete Anatomy
The brachioradialis now effectively has no ability to create rotation in this position but rather only serves to compress the joint space together. This is because its action line is roughly parallel to the bones of the forearm and its moment arm for rotation is nearly zero.
This does not, however, imply that the brachioradialis plays no role in bending the elbow in this position.
Do you recall in an earlier section when I said that these “other” flexors are just as important to the function of the biceps as the biceps themselves"?
Let's talk about why, now that we have a much better idea of how and where each of the elbow flexors function.
In position #1, we identified that the brachioradialis has a longer moment arm for rotation and therefore more leverage to create motion out of the bottom of a curl.
We also identified how the biceps and brachialis had shorter moment arms in this position and contributed a greater amount to keeping the joint space held together.
In position #2, we identified the relative changes in moment arm and its direction of influence to now favor the biceps and brachialis as opposed to the brachioradialis (in terms of rotation).
And in position #3, we identified how these changes remained the same and how the brachioradialis progressively plays more of a "joint stabilizing" role as compared to the initial position.
Do you think that it's a coincidence that our elbow flexors experience this complete shift in joint function as we move through the range of a curl?
Absolutely not! Evolution has organized muscular function in a brilliant way.
See the images below of the different joint positions now with how these action lines are complimentary to one another (I included only biceps and brachioradialis for clarity):



Credit: Compete Anatomy
Hopefully you can picture how when one muscle "stabilizes", the other(s) take on the role of creating rotation and vise-versa.
This is a mechanism of co-contraction that appears in a multitude of different locations around the body and is what allows one tissue to create high degrees of output without eroding the fabric of our joint surfaces.
Pretty cool if you ask me.
We'll talk about how these changes leverages will impact our decision-making in the gym later on, but first, let's dive into the current research that's available on the elbow flexors.